Aquatic locomotion
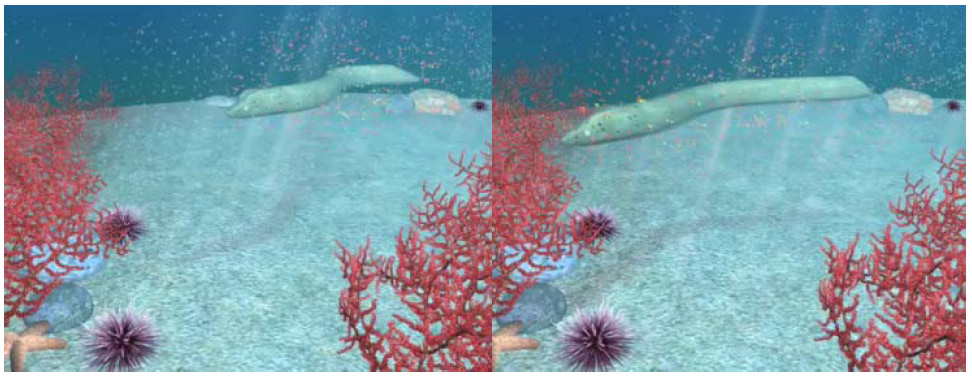
Our contributions and innovations
Flying and swimming animals utilize body and appendage/limb movements for locomotion through air and water. The physical principles underlying the extraordinary mobility of these animals have been the subject of years of effort and there is still much that is not understood. Experimental approaches are very difficult, since instrumenting these animals often disrupts the behavior of interest. Our work in this area has progressed in close collaboration with Malcolm MacIver at Northwestern University (black ghost knifefish studies), and also in collaboration with Melina Hale at The University of Chicago and David McLean at Northwestern University (larval zebrafish studies). The key contributions from our group pertaining to fundamental issues in aquatic locomotion are listed below.
I. Drag and thrust on swimming bodies
Traditional analyses of self–propulsion have centered on attempting to decompose the total force on a swimming body into drag and thrust. During steady self–propulsion, the drag and thrust would be in balance. In spite of a century of research there has been no prior instance where drag–thrust decomposition has been conclusively shown to work in case of undulatory propulsion at finite Reynolds number. In a recent work we discovered the first such instance. Our decomposition is different from that hypothesized by Lighthill, which has been widely discussed for more than four decades.
II. Hydrodynamic optimality in aquatic organisms
Lighthill and Blake had explained the peculiar morphology of gymnotiform swimmers (e.g., the black ghost knifefish; see video right) by proposing that the ability of its elongated ribbon fin to generate thrust is enhanced by the presence of the non–deforming body of the fish. This result has been widely discussed in literature over the past twenty years. We have shown that, contrary to the results by Lighthill and Blake, there is no enhancement of the propulsive force due to the presence of the body. Instead, we found that the observed height of the ribbon fin is such that the mechanical energy spent per unit distance, i.e., the mechanical cost of transport (COT), is low. In a first study of its kind we show that the optimal fin height based on COT is a direct consequence of different scalings of drag and thrust of the fin with respect to the fin height. It was also found that many other aspects of the morphology of gymnotiform swimmers are near optimal.
III. Gray’s paradox and efficiency measures in swimming
In 1936 Gray reported that the “drag” power of dolphins in the swimming direction was substantially larger than the estimates of muscle power. We revisited this “paradox” in the context of undulatory swimming. We showed that the drag power, which represents the work done on the fluid due to motion in the swimming direction, is exactly balanced by the thrust power, which represents the work done by the fluid. We also showed that during undulatory swimming most of the muscle energy is dissipated in causing the lateral motion of the body – not in overcoming the “drag” in the swimming direction. Thus, we showed that the basic premise of comparing drag power to muscle power and the related efficiency measures are not appropriate. Instead, a new non–dimensional cost–of–transport measure was proposed. This fundamental result is central to the seventy–year old debate on efficiency measures for swimming.
IV. How does muscle forcing lead to translational motion in undulatory swimming?
Swimming organisms show a variety of complex deformations during their movement. We enquired whether complex muscle forcing is required to create the observed deformation kinematics that lead to movement. We developed a novel approach where swimming is treated as a forced oscillation problem. It was shown that forcing triggers the first few fundamental deformation modes of the body – consistent with observation – which in turn drive the axial translational motion. We showed that simple forcing patterns can trigger complex looking deformation kinematics that lead to movement – a result that is critical to understand the neural control of movement.
Underwater vehicle design
The physico-mechanical designs evolved in fish are inspiring robotic devices for maneuvering and propulsion in underwater vehicles. To that end, it is crucial to understand how animals choose certain body or fin movements to vector hydrodynamic forces during propulsion and maneuvering. We intend to apply our FRS techniques to an effort led by Prof. MacIver, where he plans to build a novel highly maneuverable underwater vehicle modeled on the black ghost knifefish.
Artificial evolution
FRS techniques, coupled with genetic algorithms can lead to computational modeling of evolution (called artificial evolution) of fish forms. It could be an important tool to interpret the evolution of different types of fish and fins.
Animation
FRS of free swimming that is computationally efficient finds application in animation of fish motion. Since the fish and fluid motion is fully resolved it leads to realistic renderings not achieved to date. Previously, our FRS technique for rigid bodies was applied in animation by Carlson et al. (SIGGRAPH 2004). We are now collaborating with Prof. Bruce Gooch to develop animation of fish swimming. The image shows a snap shot of an animation based on our FRS results of a freely swimming eel.